Study Of Nucleic Acids And Proteins To Show Evolutionary Relationships
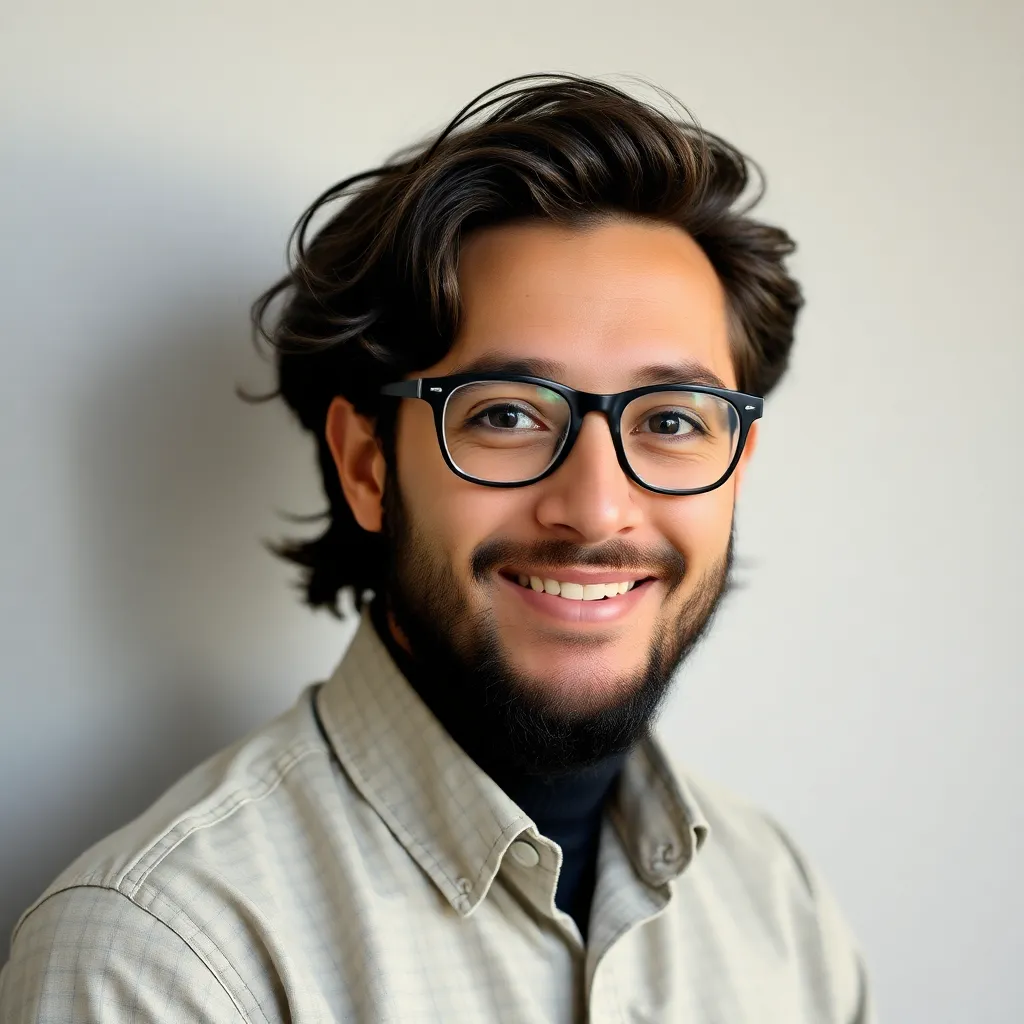
Espiral
Apr 19, 2025 · 6 min read

Table of Contents
The Study of Nucleic Acids and Proteins: Unveiling Evolutionary Relationships
The intricate tapestry of life on Earth is woven from the threads of evolution, a process that has shaped the diversity of organisms over billions of years. Understanding this process requires delving into the fundamental building blocks of life: nucleic acids and proteins. By comparing the sequences of these molecules across different species, scientists can reconstruct evolutionary relationships, illuminating the branching patterns of the tree of life and revealing the shared ancestry of all living things. This article explores the powerful tools and techniques used to study nucleic acids (DNA and RNA) and proteins to decipher evolutionary relationships, highlighting their significance in various fields of biology.
The Molecular Clock: Timekeeping in Evolution
The concept of a molecular clock is central to this field. It proposes that the rate of molecular evolution, specifically the accumulation of mutations in DNA and protein sequences, is relatively constant over time. This allows scientists to estimate the time elapsed since two species diverged from a common ancestor by comparing the number of differences in their DNA or protein sequences. However, it's crucial to acknowledge that the molecular clock isn't perfectly regular. Rates of evolution can vary due to factors like generation time, population size, and selective pressures. Despite these limitations, the molecular clock provides a valuable tool for estimating evolutionary timelines, particularly when combined with other dating methods like the fossil record.
DNA Sequencing and Phylogenetic Analysis: Tracing Evolutionary Paths
DNA sequencing, the process of determining the precise order of nucleotides (adenine, guanine, cytosine, and thymine) in a DNA molecule, is the cornerstone of modern evolutionary biology. By comparing DNA sequences from different species, scientists can identify similarities and differences, reflecting their evolutionary relationships. These sequences are then used to construct phylogenetic trees (also known as cladograms), which visually represent the evolutionary history of a group of organisms.
Different methods are employed to construct phylogenetic trees, including:
- Neighbor-joining: This method constructs a tree by iteratively joining the closest pairs of sequences.
- Maximum parsimony: This method selects the tree that requires the fewest evolutionary changes to explain the observed data.
- Maximum likelihood: This method selects the tree that has the highest probability of producing the observed data given a specific evolutionary model.
These methods, combined with powerful computational tools, allow scientists to analyze massive datasets of DNA sequences, leading to increasingly refined and accurate phylogenetic trees.
Analyzing Protein Sequences: A Complementary Approach
Proteins, the workhorses of the cell, are synthesized based on the information encoded in DNA. The amino acid sequence of a protein is a direct reflection of the underlying DNA sequence, and comparing protein sequences provides another valuable way to infer evolutionary relationships. Several advantages exist in using protein sequences:
- Slower mutation rate: Protein sequences often evolve more slowly than DNA sequences, making them particularly useful for studying ancient evolutionary events.
- Functional constraints: The functional constraints on proteins can influence their evolutionary rate, providing additional insights into the selective pressures shaping evolution.
- Conserved regions: Highly conserved regions of proteins, those that have remained virtually unchanged over vast evolutionary timescales, often play critical roles in protein function and are particularly valuable for phylogenetic analysis.
Techniques like BLAST (Basic Local Alignment Search Tool) are used to compare protein sequences and identify conserved regions. These comparisons reveal homologous proteins, proteins that share a common ancestor and often have similar functions, even across vastly different species. The degree of similarity in protein sequences provides a measure of the evolutionary distance between species.
Applications in Various Fields: Unraveling Life's History
The study of nucleic acids and proteins to infer evolutionary relationships has far-reaching implications across various fields of biology:
1. Understanding Human Evolution: Tracing Our Ancestry
By comparing human DNA sequences with those of other primates, researchers have constructed detailed phylogenetic trees depicting human evolutionary history. This work has shed light on the relationships between humans and other primates, the timing of key evolutionary events, and the genetic basis of human traits. Furthermore, analyzing ancient DNA extracted from fossils allows scientists to reconstruct evolutionary pathways, revealing migrations and interbreeding events in our past.
2. Microbial Evolution and Antibiotic Resistance: Tackling Global Health Challenges
The rapid evolution of microorganisms, especially bacteria, is a major concern, particularly in the context of antibiotic resistance. Analyzing the genetic makeup of bacteria allows researchers to track the evolution of resistance genes, understand the mechanisms driving resistance, and develop strategies to combat this growing global threat. This knowledge is crucial for developing new antibiotics and optimizing existing treatments.
3. Conservation Biology: Protecting Endangered Species
Understanding the evolutionary relationships between species is vital for conservation efforts. Phylogenetic analysis can help identify unique lineages, prioritize species for conservation, and inform strategies for managing endangered populations. By understanding evolutionary history, conservationists can better protect biodiversity and prevent extinctions.
4. Forensics: Solving Crimes Through DNA Analysis
DNA fingerprinting, a technique based on the comparison of highly variable regions of DNA, has revolutionized forensic science. By comparing DNA from crime scenes with DNA profiles from suspects, investigators can accurately identify criminals and exonerate the innocent. Understanding the variability of DNA sequences within and between populations is key to accurate DNA fingerprinting.
5. Agriculture: Improving Crop Yields and Disease Resistance
Comparing the genomes of different crop varieties can reveal genes associated with desirable traits, such as high yield, disease resistance, and drought tolerance. This knowledge can be used to develop improved crop varieties through selective breeding or genetic engineering, leading to increased food production and food security.
6. Viral Evolution and Pandemic Preparedness: Monitoring Emerging Threats
The rapid evolution of viruses, particularly RNA viruses, poses a significant challenge to public health. By analyzing viral genomes and tracking mutations, scientists can monitor the emergence and spread of viral outbreaks, predict the evolution of viral strains, and develop effective vaccines and antiviral therapies. This is especially crucial in combating emerging infectious diseases and preparing for future pandemics.
Challenges and Future Directions: Refining Our Understanding
While the study of nucleic acids and proteins has greatly advanced our understanding of evolution, several challenges remain:
- Incomplete fossil record: The fossil record provides crucial information for calibrating molecular clocks, but it remains incomplete, especially for certain lineages.
- Horizontal gene transfer: In bacteria and archaea, horizontal gene transfer, the movement of genes between organisms, can complicate phylogenetic analysis.
- Incomplete genome sequencing: For many organisms, complete genome sequences are still lacking, limiting our ability to conduct thorough phylogenetic analyses.
- Computational limitations: Analyzing massive datasets of genomic and proteomic data requires powerful computational tools and sophisticated algorithms.
Future research will likely focus on:
- Developing more accurate molecular clocks: Improving our understanding of the factors influencing molecular evolution rates is crucial for refining the accuracy of molecular clocks.
- Integrating multiple data types: Combining molecular data with other data sources, such as the fossil record and morphological data, will lead to more robust and comprehensive phylogenetic analyses.
- Developing more sophisticated phylogenetic methods: Advancing computational tools and algorithms will allow for more efficient and accurate analysis of large datasets.
- Exploring novel molecular markers: Identifying new types of molecular markers, such as those based on non-coding DNA or epigenetic modifications, will provide additional insights into evolutionary relationships.
In conclusion, the study of nucleic acids and proteins has revolutionized our understanding of evolutionary relationships. By comparing the sequences of these molecules across different species, scientists have constructed detailed phylogenetic trees that illuminate the history of life on Earth. While challenges remain, ongoing research continues to refine our understanding of evolution and its implications for various fields, from medicine and agriculture to conservation and forensic science. The power of molecular data promises further breakthroughs in our understanding of the intricate web of life and its evolutionary journey.
Latest Posts
Latest Posts
-
History Of Long Island New York
Apr 21, 2025
-
What 2 Major Agricultural Inventions Did Jethro Tull Create
Apr 21, 2025
-
The Opposite Of The Seven Deadly Sins
Apr 21, 2025
-
The Peoples Republic Of China Flag
Apr 21, 2025
-
Who Is The Father Of Sociology
Apr 21, 2025
Related Post
Thank you for visiting our website which covers about Study Of Nucleic Acids And Proteins To Show Evolutionary Relationships . We hope the information provided has been useful to you. Feel free to contact us if you have any questions or need further assistance. See you next time and don't miss to bookmark.