What Is The All Or None Principle
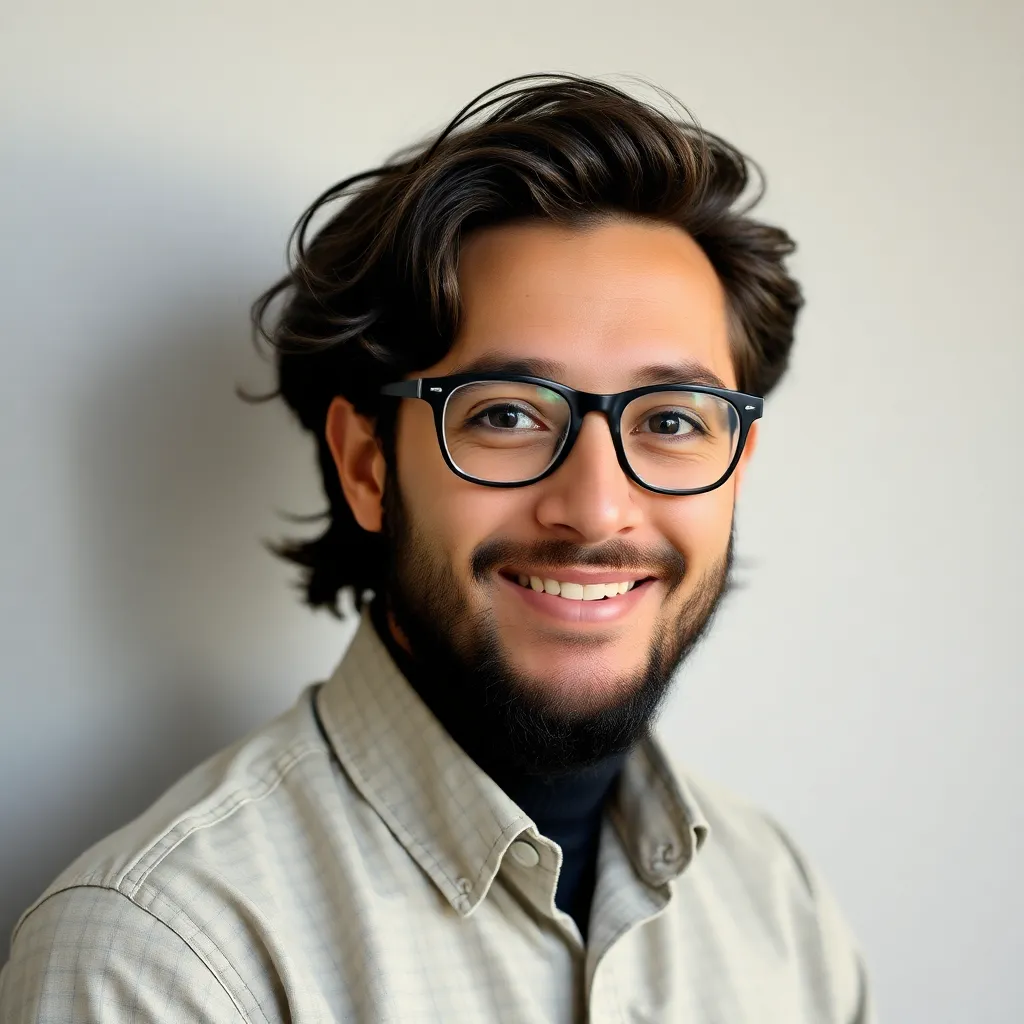
Espiral
Apr 17, 2025 · 6 min read

Table of Contents
What is the All-or-None Principle? A Deep Dive into Neural Excitation
The all-or-none principle, a cornerstone of neuroscience, dictates that the strength of a stimulus doesn't affect the strength of a response. Instead, a nerve or muscle fiber will either fire completely or not at all. This seemingly simple principle underpins a vast array of physiological processes, from muscle contractions to the transmission of sensory information. Understanding this principle is crucial for comprehending how our nervous system functions and interacts with the rest of our body. Let's delve deeper into its intricacies.
Understanding the All-or-None Principle: A Simple Analogy
Imagine a toilet flush. You can press the lever gently, or you can press it with considerable force. However, the result is the same: the toilet flushes completely. There's no such thing as a "half flush." This is analogous to the all-or-none principle. A neuron, like the toilet, either fires a complete action potential (the "flush") or it doesn't fire at all. The intensity of the stimulus (pressing the lever) doesn't change the strength of the response (the flush).
The Mechanics of Neural Excitation
To fully grasp the all-or-none principle, we must first understand how neurons transmit signals. Neurons communicate via electrical impulses called action potentials. These action potentials are triggered when the neuron's membrane potential reaches a critical threshold. This threshold is a specific voltage level. Once the threshold is reached, the neuron undergoes a rapid depolarization, where the inside of the cell becomes positively charged relative to the outside. This creates a self-propagating electrical signal that travels down the axon, the neuron's long projection.
Key Components of the Process:
- Resting Membrane Potential: Before an action potential, the neuron maintains a resting membrane potential, typically around -70 millivolts (mV). This negative potential is due to an uneven distribution of ions across the neuronal membrane.
- Stimulus: A stimulus, whether it's a sensory input, a neurotransmitter release, or another electrical signal, causes changes in the membrane potential.
- Threshold Potential: If the stimulus is strong enough to depolarize the membrane to the threshold potential (typically around -55 mV), the action potential is triggered.
- Depolarization: The rapid influx of sodium ions (Na+) into the cell causes a dramatic increase in the membrane potential, making it positive.
- Repolarization: Following depolarization, potassium ions (K+) rush out of the cell, restoring the negative membrane potential.
- Refractory Period: After repolarization, there's a brief period called the refractory period, where the neuron is unresponsive to further stimulation. This ensures the unidirectional propagation of the action potential.
The Role of Ion Channels
The all-or-none principle is intimately linked to the behavior of voltage-gated ion channels embedded in the neuron's membrane. These channels are protein pores that open and close in response to changes in membrane potential. Specifically, voltage-gated sodium channels are responsible for the rapid depolarization phase of the action potential.
When the threshold potential is reached, these sodium channels open, allowing a massive influx of sodium ions. This influx is all or none. Either enough sodium channels open to reach the threshold, resulting in a full-blown action potential, or they don't, and there's no action potential. The number of open channels determines the speed and intensity of the depolarization but does not influence the final outcome: either a full action potential or nothing at all.
How Does the Nervous System Encode Information?
If the strength of a stimulus doesn't affect the amplitude of the action potential, how does the nervous system encode information about the intensity of a stimulus? The answer lies in two primary mechanisms:
- Frequency Coding: A stronger stimulus will trigger a higher frequency of action potentials. The more frequent the firing, the stronger the perceived signal. Think of it like a machine gun; a rapid firing represents a strong signal, while slower firing represents a weaker one.
- Population Coding: A stronger stimulus might activate a larger number of neurons. The nervous system integrates the signals from multiple neurons to interpret the intensity of the stimulus. This is like having multiple machine guns firing simultaneously—the more guns, the stronger the overall effect.
The All-or-None Principle in Muscle Contraction
The all-or-none principle applies not only to neurons but also to muscle fibers. A single muscle fiber, like a neuron, will either contract completely or not at all in response to a stimulus. However, a whole muscle can produce graded contractions due to the recruitment of multiple muscle fibers. A stronger contraction involves the activation of a larger number of muscle fibers.
Exceptions and Nuances
While the all-or-none principle is a fundamental concept, it's crucial to acknowledge certain exceptions and nuances. For instance, under certain experimental conditions, graded responses can be observed in some neurons. These exceptions often involve subtle changes in the membrane potential that don't reach the threshold for a full action potential but can still influence neuronal activity.
Furthermore, the concept mainly applies to individual neurons and muscle fibers. The overall response of a tissue or organ, composed of many neurons or muscle fibers, can exhibit graded responses due to the varying degrees of activation across the population.
Clinical Significance
Understanding the all-or-none principle is vital in numerous clinical settings. For example, it helps explain the mechanism of action of certain drugs that affect nerve conduction, such as local anesthetics. Local anesthetics work by blocking voltage-gated sodium channels, preventing the propagation of action potentials and hence blocking pain signals. Similarly, diseases affecting ion channels, such as some channelopathies, can significantly disrupt nerve and muscle function.
Implications in Research and Technology
The all-or-none principle profoundly impacts neuroscience research and technological advancements. Our ability to understand neural coding, brain-computer interfaces, and the development of effective treatments for neurological disorders heavily relies on our understanding of this fundamental principle.
Conclusion: The All-or-None Principle – A Foundation of Neuroscience
The all-or-none principle, despite its apparent simplicity, is a cornerstone of our understanding of the nervous system. Its application extends from single neurons and muscle fibers to complex physiological processes and clinical implications. While nuances and exceptions exist, the principle remains a fundamental building block for comprehending how our brains and bodies process information and respond to stimuli. Its importance in research, technology and clinical practice cannot be overstated, making it a vital concept for anyone interested in the intricacies of life's fundamental processes. Further exploration into the molecular mechanisms underpinning the principle promises to yield exciting advancements in neuroscience and related fields. Understanding this principle provides a solid foundation for comprehending the complex world of neurobiology and its applications.
Latest Posts
Latest Posts
-
Where Did Neil Armstrong Live In Ohio
Apr 19, 2025
-
Selena Gomez Timeline Of Her Life
Apr 19, 2025
-
Where Is The Peru Current Located
Apr 19, 2025
-
Was Crispus Attucks A Patriot Or Loyalist
Apr 19, 2025
-
What Zodiac Sign Is February 24
Apr 19, 2025
Related Post
Thank you for visiting our website which covers about What Is The All Or None Principle . We hope the information provided has been useful to you. Feel free to contact us if you have any questions or need further assistance. See you next time and don't miss to bookmark.