Why Are Metals Good Electrical Conductors
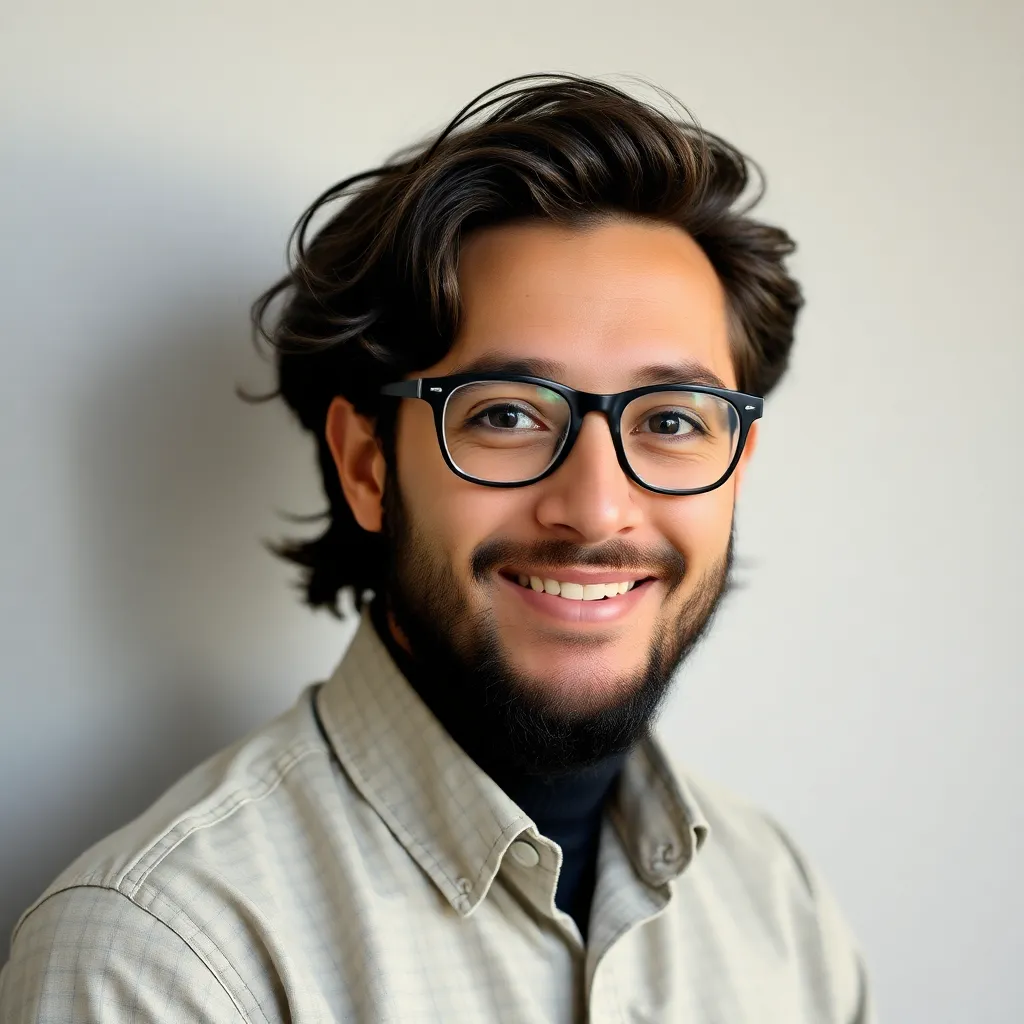
Espiral
Apr 05, 2025 · 6 min read
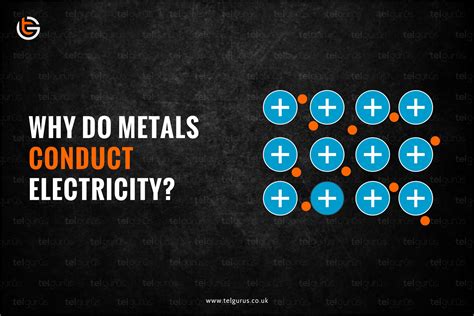
Table of Contents
Why Are Metals Good Electrical Conductors? Unraveling the Secrets of Conductivity
Metals are ubiquitous in our daily lives, from the smartphones in our pockets to the power grids that illuminate our cities. This widespread use stems, in large part, from their exceptional ability to conduct electricity. But why are metals such efficient electrical conductors? The answer lies deep within their atomic structure and the fascinating behavior of electrons. This article delves into the microscopic world to explain this fundamental property, exploring the concepts of atomic structure, electron mobility, and the factors influencing conductivity. We'll also look at specific examples and exceptions to solidify your understanding.
The Atomic Structure: A Foundation for Conductivity
The secret to a metal's excellent electrical conductivity lies in its unique atomic structure. Unlike other materials, metal atoms exhibit a distinctive arrangement and electron behavior:
The Electron Sea Model
The most widely accepted model to explain metallic conductivity is the electron sea model. Imagine a lattice of positively charged metal ions immersed in a "sea" of delocalized electrons. These electrons aren't bound to any specific atom; instead, they are free to move throughout the entire metal structure. This mobility is the key to electrical conductivity.
-
Delocalized Electrons: Unlike in insulators where electrons are tightly bound to their atoms, metal atoms readily contribute their valence electrons (outermost electrons) to this shared electron sea. This leaves behind positively charged ions, forming a stable crystalline structure.
-
Free Electron Movement: These delocalized electrons are not stationary; they are constantly in motion, moving randomly within the metal lattice. This random movement is crucial for understanding the response of metals to an external electric field.
Band Theory: A More Sophisticated View
While the electron sea model provides a simplified yet effective explanation, a more accurate and sophisticated description comes from band theory. This theory describes the energy levels of electrons in a solid. In metals, the valence band (the energy levels occupied by valence electrons) overlaps with the conduction band (the energy levels available for electron movement). This overlap allows electrons to readily transition to higher energy levels and move freely, contributing to high conductivity.
-
Valence and Conduction Bands: The energy difference between the valence and conduction bands determines the material's conductivity. In insulators, this gap is large, preventing electron movement. In semiconductors, the gap is smaller, allowing for some conductivity, while in metals, the bands overlap, resulting in extremely high conductivity.
-
Fermi Level: The Fermi level represents the highest occupied energy level at absolute zero temperature. In metals, the Fermi level lies within the conduction band, indicating a high density of free electrons ready to contribute to current flow.
The Mechanism of Electrical Conduction in Metals
When an electric field is applied across a metal, the delocalized electrons are no longer moving randomly. Instead, they experience a net drift in the direction of the electric field, creating an electric current.
Drift Velocity vs. Random Motion
It's important to distinguish between the random thermal motion of electrons and their drift velocity under an electric field. Random motion is chaotic and contributes little to net current flow. The drift velocity, on the other hand, is a slow, directed movement superimposed on the random motion, and this directed movement constitutes the electric current.
Ohm's Law and Resistance
The relationship between the current (I), voltage (V), and resistance (R) in a metal is described by Ohm's Law: V = IR. The resistance of a metal depends on factors such as its length, cross-sectional area, temperature, and the material itself (resistivity).
-
Resistivity: Resistivity (ρ) is a material property that quantifies how strongly a material opposes the flow of electric current. Metals have very low resistivity, indicating their high conductivity.
-
Temperature Dependence: The resistance of most metals increases with increasing temperature. This is because higher temperatures lead to increased lattice vibrations, which scatter the electrons and impede their flow.
Factors Influencing Metallic Conductivity
Several factors influence the conductivity of metals:
Temperature
As mentioned earlier, temperature plays a significant role. Higher temperatures increase lattice vibrations, scattering electrons and increasing resistance, thus decreasing conductivity. This is why superconducting materials, which exhibit zero resistance at very low temperatures, are so remarkable.
Impurities
The presence of impurities in a metal can also affect its conductivity. Impurities act as scattering centers for electrons, impeding their flow and increasing resistance. Highly pure metals generally exhibit higher conductivity than impure ones.
Crystal Structure and Defects
The arrangement of atoms in the metal lattice and the presence of defects (like dislocations or vacancies) can affect electron mobility. A well-ordered, defect-free lattice facilitates electron flow, while defects act as obstacles, increasing resistance.
Alloying
Alloying—the process of combining different metals—can significantly alter the electrical conductivity. The resulting alloy might have a higher or lower conductivity depending on the constituent metals and their proportions. Some alloys are designed to have specific electrical properties, such as high resistance for heating elements or specific conductivity for wiring applications.
Examples of Metals with High Conductivity
Copper and silver are classic examples of metals renowned for their excellent electrical conductivity. Their widespread use in electrical wiring and electronic components is a testament to their capabilities.
-
Copper (Cu): Copper's high conductivity, ductility (ability to be drawn into wires), and relatively low cost make it the most common metal for electrical wiring.
-
Silver (Ag): Silver has even higher conductivity than copper, but its high cost limits its use to specialized applications where high conductivity is paramount, such as high-frequency circuits.
-
Aluminum (Al): Aluminum is another commonly used metal in electrical applications, offering a good balance between conductivity and cost. It's lighter than copper, making it suitable for overhead power lines.
-
Gold (Au): Gold's high conductivity and resistance to corrosion make it ideal for connectors and other critical components in electronic devices.
Exceptions and Considerations
While most metals are excellent conductors, there are exceptions and nuances:
-
Transition Metals: The conductivity of transition metals is generally lower than that of alkali or alkaline earth metals due to their partially filled d-orbitals, which influence electron mobility.
-
Alloys: As previously mentioned, the conductivity of an alloy can vary significantly depending on its composition.
-
Semiconductors and Insulators: While not metals, semiconductors and insulators provide a stark contrast to the behavior of metals concerning electrical conductivity. Their band structures prevent or restrict free electron movement, leading to vastly lower conductivities compared to metals.
Conclusion: The Electrifying World of Metallic Conductivity
The remarkable electrical conductivity of metals arises from their unique atomic structure and the presence of a sea of delocalized electrons. These electrons, free to move throughout the metal lattice, readily respond to an applied electric field, establishing an electric current. This fundamental property is the cornerstone of countless technological advancements, powering our modern world. Understanding the underlying principles of metallic conductivity allows us to design and optimize materials for a wide range of applications, from large-scale power transmission to the intricate circuitry of microchips. The ongoing exploration of new materials and alloys continues to refine our understanding and push the boundaries of electrical conductivity in pursuit of technological progress.
Latest Posts
Latest Posts
-
Me And My Sister Or My Sister And I
Apr 05, 2025
-
Male And Female Reproductive System Chart
Apr 05, 2025
-
What Does Venezuelas Flag Look Like
Apr 05, 2025
-
What Is Said About The Antichrist
Apr 05, 2025
-
In Search Of Lost Time Summary
Apr 05, 2025
Related Post
Thank you for visiting our website which covers about Why Are Metals Good Electrical Conductors . We hope the information provided has been useful to you. Feel free to contact us if you have any questions or need further assistance. See you next time and don't miss to bookmark.